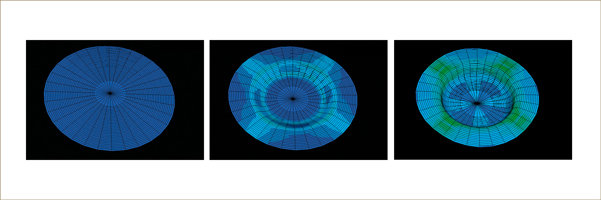
Cooperation with Fraunhofer
Within the framework of the Pact for Research and Innovation, the Max Planck Society and Fraunhofer-Gesellschaft intend to continue and intensify their cooperation across research areas and disciplines. With its focus centred on application, the collaboration with Fraunhofer-Gesellschaft is of particular interest to the Max Planck Society. Against this background, the two organizations have been engaged in talks since spring 2004 in order to identify and support collaboration opportunities at the interface of application oriented research and basic research. This includes meanwhile the fields of computer science, materials science / nanotechnology and biotechnology, as well as the area of regenerative energies and photonics. The aim of such a venture is to bring to application the knowledge resulting from collaborative efforts, thereby making a direct contribution to the development of new technologies.
RICIMER – Roman-inspired concrete innovation by multi-analytical enhanced Research
A building material from ancient Rome continues to set the benchmark to this very day. The Romans used self-healing, earthquake-proof concrete to build structures such as the dome of the Pantheon by mixing in volcanic ash rather than Portland cement. This method generates far fewer CO2 emissions than the concrete produced today. The Ricimer team now wants to re-discover the formula for this building material, which was lost in the Middle Ages. One reason for this is that volcanic ash is similar to the melt products from municipal incineration ashes, which could be turned into a useful product in concrete production. By developing alternative building materials, the researchers want to help establish a sustainable closed-loop economy and reduce global CO2 emissions. To successfully use the slag from incineration plants to replace cement, the researchers will first take a closer look at the complex microstructure of Roman concrete and investigate which factors influence the structure and give the concrete its self-healing and other properties. This research should then provide an insight into how slag needs to be processed to create concrete similar to the building material used in ancient times.
Participating Institutes:
Fraunhofer Institute for Building Physics – IBP
Max Planck Institute for Solid State Research
Duration 2022 – 2026
LAR3S – Laser-generated, three-dimensional photonic components
Optical data exchange and processing are becoming increasingly widespread. What's more, the success of quantum information processing is reliant on the availability of photonic components that can transport, shape, store, and filter light signals with high spectral selectivity. These kinds of components are also useful for spectroscopic techniques in basic research and optical sensors. To make this a reality, the partners in the LAR3S project are developing methods to manufacture microresonators and photonic-crystal fibers (PCF) that will open the door to new possibilities in optical signal processing. Microresonators are annular components that can store and modulate light, which researchers now want to manufacture by combining the selective laser-induced etching method with laser polishing. The goal is to create resonators that are better able to store light and use other non-annular geometries, such as in the form of a Möbius strip or the figure eight, to allow new functions to be integrated when processing light. Besides this, the researchers are also looking to improve and enhance the properties and functions of PCFs by using a new manufacturing method. PCFs use cavities in a fiber-optic core to guide and modulate light. Researchers now want to manufacture the glass rods from which the fibers are drawn by drilling the cavities in a fused silica rod using the inverse laser drilling technique instead of laboriously assembling the precursors of the fibers from glass capillaries. This will make it possible to create PCFs with new geometries and new properties.
Participating Institutes:
Max Planck Institute for the Science of Light
Fraunhofer Institute for Laser Technology ILT
Fraunhofer Institute for Silicate Research ISC
Duration: 2022 – 2025
GT-4-ET – Glass technologies for the Einstein telescope
The detection of gravitational waves in 2015 opened up a whole new world of sensory impressions in astronomy. While it was previously only possible to explore visible cosmic processes, events can now, in a sense, also be heard through tiny distortions in spacetime. This includes phenomena that are otherwise invisible. But it takes very keen ears to pick up these extremely weak gravitational waves. The researchers from the GT-4-ET project want to help significantly improve the hearing of the planned Einstein Telescope in comparison to current gravitational wave detectors. One approach is to cushion the detector even better against vibrations in the ground. To achieve this goal, the team is developing sensors that will provide extremely precise measurements of the movements of the suspended, roughly 200-kilogram, glass mirror. These movements will then be actively suppressed. What's more, GT-4-ET is targeting a new method for measuring tiny fluctuations in laser power and directly compensating for these fluctuations in an integrated system. This will allow the researchers to reduce the noise generated by the detector, which can currently only detect particularly strong signals. Both the motion sensors and noise suppression require the use of new technologies to manufacture the sensors as well as other elements. These technologies could then have applications beyond gravitational wave astronomy in other areas that demand extremely high precision, such as in other fields of astronomy as well as drilling equipment in mining or quantum communication.
Participating Institutes:
Max Planck Institute for Gravitational Physics (Albert Einstein Institute)
Fraunhofer Institute for Applied Optics and Precision Engineering, IOF
Duration: 2022 – 2026
AutoRAPID – High-throughput screening platform for physical phenotyping of cells
The difference between a healthy and sick cell can often also be determined by how easily it can be deformed. Tumor cells, for instance, are softer than healthy cells. The researchers in this project have exploited this attribute to establish new diagnostic methods. The Max Planck partners have invented real-time deformation cytometry, which uses optical measurements to measure the biomechanical properties of between 100 and 1,000 cells a second. The colleagues at the Fraunhofer Institute will then use their skills in process automation for life sciences to build on this foundation to develop fully-automatic processes that will enable the analysis of more than 100 samples a day without any expert knowledge. The researchers will also integrate further optical analysis methods to provide even more information and machine learning will be used to help interpret the data. The capability of the optimized processes will then be demonstrated in a comprehensive study that will investigate how thousands of medicinal substances influence the biomechanical properties of cells.
Participating Institutes:
Fraunhofer Institute for Manufacturing Engineering and Automation (IPA)
Max Planck Institute for the Science of Light
Duration:
2021 – 2024
NeuroHum - Neurocognitively-guided modeling of virtual humans for enhanced realism in immersive media
Getting something almost right is often scant comfort. There is not much use in virtual humans almost looking real. After all, that's when they have a more unsettling effect than a stylized cartoon character. Better knowledge of which attributes of an avatar cause this uncomfortable feeling should help to create realistic virtual humans. These could then be used in areas such as animated films, computer games, and immersive media with virtual or augmented reality in which users can immerse themselves. Which attributes of the face, gestures, or posture and movement do computer graphic artists need to devote more attention to in future? To answer this question, the project partners will first conduct neurocognitive experiments using virtual reality to analyze how the human brain reacts to certain differences from a photorealistic figure. This will also teach them more about how we perceive people under natural conditions.
Participating Institutes:
Fraunhofer Institute for Telecommunications, Heinrich Hertz Institute (HHI)
Max Planck Institute for Human Cognitive and Brain Sciences
Duration: 2021 – 2025
CarboGels – Carbon–Xerogel materials for electrical energy storage systems
Energy transition and electromobility will be possible only with improved energy storage systems. Whether and how quickly we can do without fossil fuels depends on how efficiently and cost-effectively we can store sustainably generated energy in batteries. The research team of the CarboGels project aims to use the previously overlooked carbon–xerogel materials (CarboGels) in batteries. This is because the stable carbon materials with a particularly large number of fine pores combine the advantages of various substances that have so far been used as electrode materials in energy storage systems. They are more conductive than previous low-cost solutions and considerably less expensive than particularly high-performance materials such as graphene. The scientists in the project want to produce CarboGels on a pilot scale and use them in a redox flow battery. They also want to further improve the properties, occupational safety, and environmental compatibility of the material. In order to one day be able to use CarboGels on a large scale, the team is also creating a concept for an industrial production process with the lowest possible costs.
Participating Institutes:
Fraunhofer Institute for Environmental, Safety, and Energy Technology UMSICHT
Max-Planck-Institut für Kohlenforschung
Duration: 2020 – 2024
Snifits4Health – Novel platform technology to revolutionize clinical chemistry and medical diagnostics
The medicine of the future will be personalized. Whether the dosage of antibiotics administered in hospital or a therapy that must be constantly adapted to the course of the disease: soon, both diagnostics and therapy will be individually tailored to each patient so that the treatment will be as successful as possible. The aim of the Snifits4Health project is to replace time-consuming laboratory tests and be able to analyse blood samples quickly and on site. The researchers of the project are developing a simple medical test kit that can also be operated by the patients themselves. At its core is a microfluidic chip that is equipped with a biosensor. To do this, the Snifits4Health team combines knowledge from microfluidics, optics, synthetic chemistry, and protein engineering. The microfluidic chip is used as a carrier on which a drop of the patient’s blood is combined with a bioluminescent sensor protein. When the sensor protein interacts with an active ingredient or biomarker of a particular disease in the blood, the colour of the light emitted by the protein changes. The biosensor is specifically tailored to a certain target – for example an antibiotic – and the colour of the light changes depending on the concentration of this target substance in the blood. This allows doctors and patients to easily obtain precise information about the current state of health with the help of a small reading device and to adjust the therapy on an individual basis.
Participating Institutes:
Fraunhofer Institute for Cell Therapy and Immunology IZI
Fraunhofer Institute for Applied Optics and Precision Engineering IOF
Max Planck Institute for Medical Research
Duration: 2020 – 2023
eBIOCO2 – Producing special chemicals through electrically powered CO2 conversion
Crude oil remains the chemical industry’s most important raw material, from which plastics, paints and components of medicines are produced. The aim of the eBIOCO2 Project is to replace at least a part of the fossil fuel with CO2 for a circular economy that will reduce chemical production’s carbon footprint. The researchers aim to use CO2 for the production of various chemical products. With the help of electricity from wind and water power or photovoltaics, they plan to introduce the greenhouse gas into synthetic biochemical processes that recreate natural photosynthesis. For this they combine approaches from biochemistry, enzyme biology and synthetic biology, developing bio-electrodes to use electricity to drive enzymes that will work together to transform CO2 into usable chemical substances. The scientists will optimize this synthetic enzyme cascade with the aid of synthetic biology so that the process runs as efficiently as possible. To bring the project to a close, they will build a demonstrator to produce the amino acids alanine, glycine and aspartate from CO2 in order to prove the viability of electrically powered biocatalytic CO2 conversion.
Participating Institutes:
Fraunhofer Institute for Interfacial Engineering and Biotechnology
Max Planck Institute for Terrestrial Microbiology
Duration: 2019 – 2023
NeurOpto – optogenetic nerve cell stimulation for medical implants
Artificial hearing aids – cochlear implants – stimulate the auditory nerve by means of tiny electrodes and can thus give the hearing impaired at least some hearing. Because the electrodes excite a wide range of frequencies, the brain has difficulty distinguishing between acoustic signals with similar frequencies. Cochlear implant users can therefore usually understand speech only when in a quiet environment. They can hardly perceive the melodies in speech and music. Optogenetic implants could offer an alternative to conventional prostheses. Neuroscientists can use it to switch individual nerve cells equipped with a light-sensitive ion channel – channel rhodopsin, which originates from unicellular algae – on and off in a targeted manner using light. The researchers want to show that optical stimulation by means of organic light-emitting diodes (OLEDs) can activate nerve cells strongly enough even in living organisms. They also want to create an interface that can both emit and detect light. This would allow the efficiency of the stimulation to be measured and the intensity to be individually adapted to the patient. Intelligent implantable stimulators based on optical stimulation could also be used for other medical therapies such as laryngeal pacemakers, cardiac pacemakers, pain control, and deep brain stimulation.
Participating Institutes:
Fraunhofer Institute for Organic Electronics, Electron Beam and Plasma Technology FEP
Max Planck Institute for Multidisciplinary Sciences (City Campus)
Duration: 2020 – 2022
RASCAL – Racetrack memory scaling for ultra-dense and energy-efficient data storage
Worldwide, increasingly more space and energy are needed to store data. The prosperity and security of our society are increasingly based on the processing and storage of large amounts of data. Because conventional hard drives are reaching their limits, the researchers of the RASCAL project want to use new findings in spintronics to establish a new storage technology. A Racetrack Memory promises a 100-fold increase in memory capacity with higher write and readout speeds. It will also work more reliably and consume less power. Spintronics uses the magnetic moment of the electron to represent and process information. The core of the new storage medium will be a magnetic nanowire on which the information will be stored in magnetic compartments – or, more precisely, in their boundaries (present/absent). This requires integrated stationary read/write heads. When a spin current is applied to the wire, the boundaries move on the wire like remote-controlled cars on a racetrack. When they pass the read/write head, data can be either stored or read. In recent years, advances have been made in racetrack memories large enough to study with optical methods. In the next step, the researchers want to make the wires smaller and integrate the read-write heads. In this way, the RASCAL team wants to create a fast and powerful storage medium that could soon be used on a large scale.
Participating Institutes:
Fraunhofer Institute for Laser Technology IPMS
Max Planck Institute of Microstructure Physics
Duration: 2020 – 2022
TWISTER – Turbulent weather in structured terrain
Clouds still pose a great many questions for climate researchers and meteorological services. The researchers in the TWISTER Project are investigating when clouds form, the conditions in which they form precipitation, and the conditions in which they then disperse. It is important for local weather forecasts, not least for extreme weather warnings, to be able to reproduce these processes in models. One thing is for sure – turbulent air plays a pivotal role in cloud physics. Researchers have not yet been able to measure these processes properly, however, as circulation measuring kilometres or several hundreds of metres jostle with turbulence that measure mere millimetres. The structure of the ground also has a major influence on local weather events, and not only mountains, over which clouds often gather, but also street canyons in cities, for example. The TWISTER researchers are now developing a LiDAR system to disperse three-dimensionally flows of up to one cubic metre, and to measure the air temperature and humidity. For this, they are employing three synchronized lasers that will determine the various physical quantities with different colours. Such a LiDAR device could be used for an array of atmospheric studies. In the TWISTER Project, the researchers complement the LiDAR investigations with detailed analyses of cloud microphysics, for which they launch a balloon with measurement devices into what are for them, interesting parts of a cloud. They then develop models from the data collected, taking into consideration the effects in various dimensions.
Participating Institutes:
Fraunhofer Institute for Physical Measurement Techniques
Max Planck Institute for Dynamics and Self-Organization
Duration: 2019 – 2022
ClusterBatt – Metal cluster formation in carbon materials – Safe anodes for cyclically stable batteries with high power density
In order for electricity to come principally from renewable sources in the future, high-performance storage facilities will be necessary – for example, batteries that use readily available raw materials. These will also be required if electric cars are to increasingly replace conventional vehicles. In the ClusterBatt Project, researchers are developing materials for lithium or sodium batteries to fulfil this requirement. More specifically, they are looking at the anode, the negative pole of the electricity storage mechanism. As no satisfactory solution has yet been found, battery performance is still significantly limited. The ClusterBatt researchers are therefore counting on carbon with microscopic pores, and they will precisely adjust the size and shape of these. It is in these pores that the lithium, or in the future sodium too, will gather while charging in the form of clusters, or tiny grains. The anodes in conventional lithium batteries consist of carbon, but in the form of graphite, where the metal atoms are deposited separately between the layers. This is why current carbon anodes are not capable of reaching high storage densities. Anodes made of pure metal or an alloy are markedly superior to them in this respect, but cannot be charged and discharged as often, and have a tendency to short-circuit because the metals do not separate them in a controlled way, instead forming branches to the cathode. Through the combination of porous carbon with metal clusters, the researchers now plan to connect the advantages of the materials currently used without having to accept their drawbacks.
Participating Institutes:
Fraunhofer Institute for Material and Beam Technology
Fraunhofer Institute for Chemical Technology
Max Planck Institute of Colloids and Interfaces
Duration: 2019 – 2022
Acoustograms – A new approach for 3D ultrasound
Ultrasound is used in a wide variety of applications; from simple sensory systems such as for example distance measurement in a car, all the way to costly imaging processes in medicine. The sound fields necessary have been created up to now with lots of individual sound converters. However, each of these requires its own electrical activation, which on the one hand makes them expensive, and on the other complicates miniaturization. Miniaturization, would certainly be of interest for many medical applications, such as for example updated endoscopy processes. In order to solve this problem, the researchers in the Acoustogram Project have developed a process that produces sound fields similar to a hologram producing a light field that transmits a three-dimensional impression. A static phase plate forms a sound field with the help of a structured surface: Sound needs different amounts of time to traverse areas of different thickness. The researchers calculate individually how a phase plate has to be structured for each sound field. To make the method ready for practical applications and to generate three-dimensional sound fields, the team of scientists are developing efficient processes to identify the sound fields as well as the structure of the phase plates that produce these sound fields. In this, they rely on methods of machine learning. They also aim to exploit the possibilities of acoustograms to make current ultrasound techniques for medicine more compact and precise.
Participating Institutes:
Fraunhofer Institute for Biomedical Engineering
Max Planck Institute for Intelligent Systems, Stuttgart
Duration: 2018 – 2022
Glyco3Display – Sugar-DNA combi-molecules as new anti-microbial agents
To facilitate the search for sugar-based agents against pathogens and to identify appropriate substances – these are the objectives of the Glyco3Display Project. Polysaccharides, also known as glycans, are intended to block the proteins on the surface of bacteria and viruses with which the microbes latch onto and penetrate cells in our bodies. To identify such substances, the researchers produce many different glycans with an efficient method that they have already developed. They affix the polysaccharides to DNA strands that are folded into a rigid frame as in the Japanese art of origami. The sugar molecules are positioned at a determined distance from one another on the DNA frames. In this way, researchers emulate how glycans are arranged on the surface of cells. The scientists will start by testing the connections between DNA and different sugars in dissolved form. In this, with the help of dye molecules that they will fasten to the DNA frames and the ends of the sugars, they will also analyse whether the sugars in the solution are bent or stretched. This will provide clues about the structure that actually connects the pathogen proteins. The researchers will also affix the sugars with and without DNA to chips using a method developed in-house, in order to investigate many different candidates in parallel. Glycans that prove themselves in the tests will then also be tested as antibodies against pathogens in animals.
Participating Institutes:
Fraunhofer Institute for Cell Therapy and Immunology
Max Planck Institute of Colloids and Interfaces
Duration: 2018 – 2022